Where to Wear It : Wearable Technology BODY MAPS
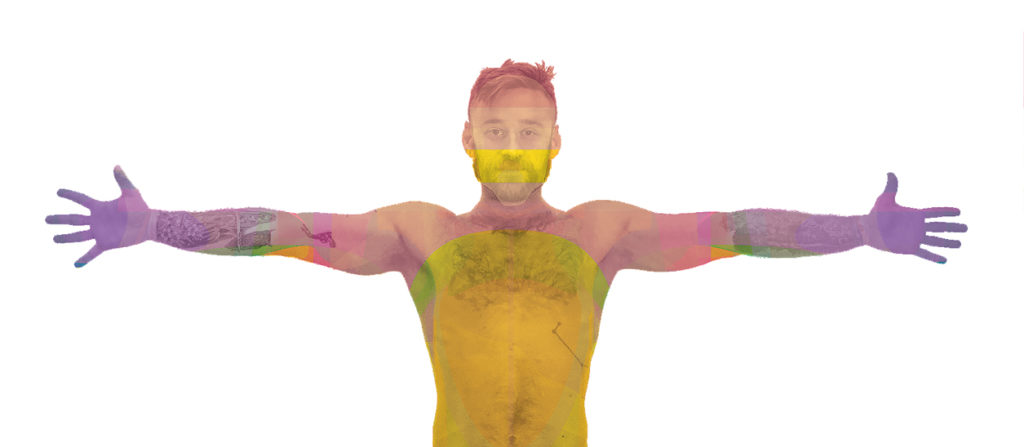
For use of content or Body Maps in acdemic publications
Please Cite:
The contents of this web page were developed under a grant from the National Institute on Disability, Independent Living, and Rehabilitation Research (NIDILRR grant number 90RE5025). NIDILRR is a Center within the Administration for Community Living (ACL), Department of Health and Human Services (HHS). The contents of this web page do not necessarily represent the policy of NIDILRR, ACL, HHS, and you should not assume endorsement by the Federal Government.
Designers may use these BODY MAPS to help determine where on the body they should place wearable technology. The maps and guidelines are free to use, and are organized by different sensing needs and human body affordances. Clint Zeagler and the Georgia Tech Wearable Computing Center retains the copyright to all images and diagrams on this page. Use with citation and photo credit.
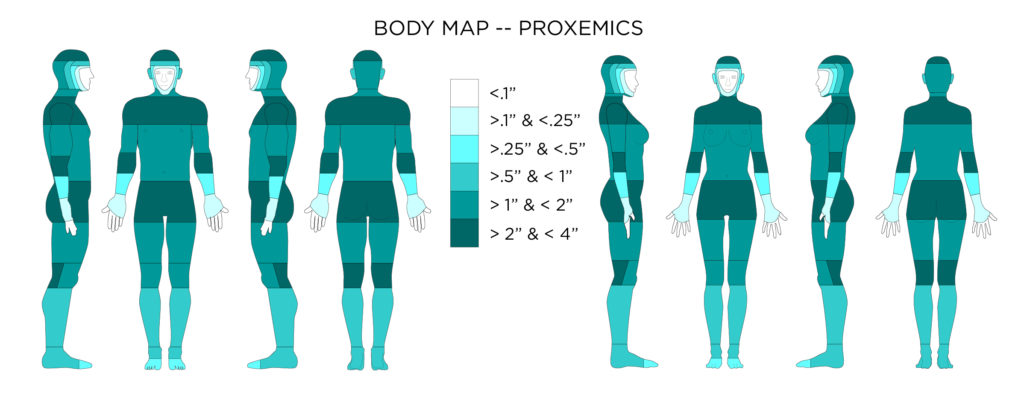
Proxemics as defined here is a human’s perception of self-size. The distance from the body portrayed on this body map indicates how far from the body a wearable device might extend and still be naturally considered part of the person’s self-size awareness. Items extending beyond this distance from the body might take a period of time for a person to adjust and account for the object within their personal self-size envelope.
Design Considerations for Proxemics
- If a wearable device or garment extends beyond the wearer’s self perceived body size then the device or garment will obstruct natural movement within the environment. There will be a period of adjustment (through continued use) before the wearable device is incorporated into a person’s perceived size of self.
- Some parts of the body can accommodate larger wearable devices without the protrusion from the body extending beyond a person’s perceived size of self.
Accessibility Considerations for Proxemics
- Someone with a body limitation that requires the use of a wheel chair (or other assistive device) may have a much different self-perceived size that would include their assistive device, and normal posture.
- Attachments to a required assistive device will also affect proxemics, and should be viewed as a “wearable”
[1] Gemperle, F., Kasabach, C., Stivoric, J., Bauer, M. and Martin, R. 1998. Design for wearability. Digest of Papers. Second International Symposium on Wearable Computers (1998).
[2] Hall, E.T. 1990. The Hidden Dimension.
[3] Stein, R., Ferrero, S., Hetfield, M., Quinn, a. and Krichever, M. 1998. Development of a commercially successful wearable data collectionsystem. Digest of Papers. Second International Symposium on Wearable Computers (Cat. No.98EX215). (1998).
[4] Tiley, A.R. and Henry Dreyfuss Associates 2001. The Measure Of Man And Woman: Human Factors In Design. John Wiley & Sons.
This body map shows the amount of weight, or pressure that can be placed on the area before the pressure becomes a discomfort.
Design Considerations for Weight Distribution
- Weight, Load, or the pressure of weight should be placed on the fleshy, but non-sensitive parts of the body, avoiding boney areas.
- The lower waist is a good area for heavy loads.
- Weight should be balanced across the body evenly, and aligned to the center of gravity is possible.
- Heavy items should not be placed on the body’s extremities for long periods of time.
- Batteries for wearable device tend to be the source of most of the weight. If a device needs a large battery (to last a long time or because it needs large amounts of power to function) place the large battery on the waist. If the wearable needs to be located on a different part of the body for use then consider distributing the power from the area of use. Finally consider distributing battery cells instead of using one large battery.
- From a design perspective weight also has a visceral quality. Density, or heaviness compared to size, in combination with other material aspects such as metallic textures are perceived to be luxurious. Donald Norman explains, “Physical objects have weight, texture, and surface. Physical feel matters. We are after all, biological creatures, with physical bodies, arms, and legs.” (D. Norman 2005) Use weight where appropriate to create a positive experience with the wearable technology object or garment.
Accessibility Considerations for Weight Distribution
- Watkins states: “One aspect of Load Analysis to consider is that even though these tests provide data on pressure levels, not all individuals or areas of the body respond in the same way to pressure. Age, sex, medical conditions and other factors may affect the way in which pressure affects mobility.” (Watkins 1995)
- Of course its easy to assume that designers want the most light weight wearable technology anyway, but its good to also remember that being light weight can make the wearable technology useful to broader communities (elderly, arthritic patients, children etc.)
[1] Gemperle, F., Kasabach, C., Stivoric, J., Bauer, M. and Martin, R. 1998. Design for wearability. Digest of Papers. Second International Symposium on Wearable Computers (1998).
[2] Scribano, F., Bruns, M. and Baron, E.R. 1970. DESIGN , DEVELOPMENT AND FABRICATION OF A PERSONNEL ARMOR LOAD PROFILE ANALYZER.
[3] Sullivan, S. 2016. Designing for Wearables: Effective UX for Current and Future Devices. O’Reilly Media.
[4] Watkins, S.M. 1995. Clothing: The Portable Environment. Iowa State Press.
[5] Zeagler, C., Starner, T., Hall, T. and Wong Sala, M. 2015. Meeting the Challenge: The Path Towards a Consumer Wearable Computer. Georgia Institute of Technology.
This body map shows the best places to put wearable devices on the body, where they will be the least obtrusive and cause the least amount of body motion impedance.
Design Considerations for Body Motion
- Large, bulky, or rigid objects should not be placed on the inside of joints, or the concave areas where the body bends.
- Rigid objects, or flexible but non-elastic objects should not be adhered to the outside of joints in a way that hinders the skin on the outside of the joint from stretching.
- Smart garments, clothing, or e-textiles should have ample room, or flexible and elastic properties to allow all parts of the body to move effectively.
- Larger, or rigid objects should be located in zones on the body with relatively limited movement or linkages.
Accessibility Considerations for Body Motion
- All body movement criteria should apply the same to individuals of impaired self-movement, unless the wearable is specifically designed to stabilize the body for medical purposes.
- Individuals may be unable to feel or move parts of their body, but these body parts still have the capability of movement from outside sources. This means that a discomfort from an inappropriately placed wearable device will not be felt, and could cause harm from extended wear.
[1] Gemperle, F., Kasabach, C., Stivoric, J., Bauer, M. and Martin, R. 1998. Design for wearability. Digest of Papers. Second International Symposium on Wearable Computers (1998).
[2] Roebuck, J. 1968. A system of notation and measurement for space suit mobility evaluation. Human Factors. 10, l (1968), 79–94.
[3] Tiley, A.R. and Henry Dreyfuss Associates 2001. The Measure Of Man And Woman: Human Factors In Design. John Wiley & Sons.
[4] Watkins, S.M. 1995. Clothing: The Portable Environment. Iowa State Press.
For sensing whole body motion, and limb motion accelerometers, gyroscopes, and magnetometers can be used at locations indicated. For sensing joint movement flex or stretch sensors can be used at locations indicated. Force and explain the impact of movement. If trying to capture movement within an environment, magnetometers (for direction) and barometric pressure sensors (for elevation change) may be used.
Design Considerations for Movement Sensor Placement
- If trying to capture whole body motion accelerometers, gyroscopes, and magnetometers should be placed close to the center of gravity on the chest.
- If trying to capture motion of limbs accelerometers and gyroscopes can be placed on the limbs. Combinations of sensors in lower and upper limb configurations can aid in more defined movement capture (such as bending of joints, and gate).
- If trying to capture motion with respect to the environment magnetometers (for direction) and barometric pressure sensors (for elevation change) may be used.
- Bend / Flex sensors can be used to determine joint bends and degree of bending. These sensors should be placed across the joint so the movement of the joint cause the sensor to bend. Bend / Flex sensors are often used in gloves to detect finger movement, and in sleeves to detect elbow movement.
- Stretch sensors can be used in the same way as bend sensors. When sewn into form fitting garments properly stretch sensors can give most of the same information bend sensors can, without the added rigidity from the sensor housing.
- Force sensors are often used in shoe applications to detect steps and also a number of other movements by detecting the force placed on different parts of the foot. Force sensor placed in glove fingertips can tell tapping and pressing. This information combined with other sensing information can give a very complete picture of body movement.
Accessibility Considerations for Movement Sensing
- On-body motion/movement sensors can be a great noninvasive way to monitor someone who has a disability or is “aging in place”. Because the movement sensing can be complete and complex, but also can be done without respect to location there is a level of privacy.
- Depending on body-placement of the movement sensors whole body motion, or specific body motion can be obtained. If monitoring someone for mobility a whole body movement sensor placed at the chest might be used to see if an aging person is “getting around”. Where as if a person is in rehabilitation from knee surgery, perhaps a movement sensor might be used to see if they are complying with their exercises.
[1] Accelerometer Basics: https://learn.sparkfun.com/tutorials/accelerometer-basics. Accessed: 2017-01-17.
[2] Aminian, K. and Najafi, B. 2004. Capturing human motion using body-fixed sensors: Outdoor measurement and clinical applications. Computer Animation and Virtual Worlds. 15, 2 (2004), 79–94.
[3] Aminian, K., Rezakhanlou, K., De Andres, E., Fritsch, C., Leyvraz, P.F. and Robert, P. 1999. Temporal feature estimation during walking using miniature accelerometers: an analysis of gait improvement after hip arthroplasty. Medical & biological engineering & computing. 37, (1999), 686–691.
[4] Barometric Pressure Sensor Hookup Guide: https://learn.sparkfun.com/tutorials/t5403-barometric-pressure-sensor-hookup-guide?_ga=1.193357220.804409743.1473347658. Accessed: 2017-01-17.
[5] Byrne, C.A., Rebola, C.B. and Zeagler, C. 2013. Design research methods to understand user needs for an etextile knee sleeve. Proceedings of the 31st ACM international conference on Design of communication – SIGDOC ’13. (2013), 17.
[6] Conductive Rubber Cord Stretch Sensor: https://www.adafruit.com/products/519. Accessed: 2017-01-17.
[7] Farringdon, J., Moore, A.J., Tilbury, N., Church, J. and Biemond, P.D. 1999. Wearable sensor badge and sensor jacket for context awareness. Digest of Papers. Third International Symposium on Wearable Computers. (1999), 107–113.
[8] Flex Sensor Hookup Guide: https://learn.sparkfun.com/tutorials/flex-sensor-hookup-guide?_ga=1.193514788.804409743.1473347658. Accessed: 2017-01-17.
[9] Force Sensitive Resistor Hookup Guide: https://learn.sparkfun.com/tutorials/force-sensitive-resistor-hookup-guide?_ga=1.234971272.804409743.1473347658. Accessed: 2017-01-17.
[10] Godbout, A. and Boyd, J.E. 2010. Corrective sonic feedback for speed skating: a case study. Proceedings of the 16th International Conference on Auditory Display (ICAD 2010). (2010), 23–30.
[11] Gyroscope: https://learn.sparkfun.com/tutorials/gyroscope/how-a-gyro-works. Accessed: 2017-01-17.
[12] Hernandez, J. and Picard, R.W. 2014. SenseGlass: Using Google Glass to Sense Daily Emotions. Proceedings of the adjunct publication of the 27th annual ACM symposium on User interface software and technology – UIST’14 Adjunct. (2014), 77–78.
[13] Imogen Heap’s Mi.Mu gloves will “change the way we make music”: 2014. https://www.youtube.com/watch?v=ci-yB6EgVW4. Accessed: 2017-01-13.
[14] Ishimaru, S., Kunze, K. and Kise, K. 2014. In the Blink of an Eye – Combining Head Motion and Eye Blink Frequency for Activity Recognition with Google Glass. Ah. (2014), 15:1-15:4.
[15] Karantonis, D.M., Narayanan, M.R., Mathie, M., Lovell, N.H. and Celler, B.G. 2006. Implementation of a real-time human movement classifier using a triaxial accelerometer for ambulatory monitoring. IEEE Transactions on Information Technology in Biomedicine. 10, 1 (2006), 156–167.
[16] Kramer, J.F. 2000. ACCURATE, RAPID, RELIABLE POSITION SENSING USING MULTIPLE SENSING TECHNOLOGIES. 6,148,280. 2000.
[17] Kuo, Y.L., Culhane, K.M., Thomason, P., Tirosh, O. and Baker, R. 2009. Measuring distance walked and step count in children with cerebral palsy: An evaluation of two portable activity monitors. Gait and Posture. 29, 2 (2009), 304–310.
[18] Lindemann, U., Hock, A., Stuber, M., Keck, W. and Becker, C. 2005. Evaluation of a fall detector based on accelerometers: A pilot study. Medical and Biological Engineering and Computing. 43, 5 (2005), 548–551.
[19] Liszka-Hackzell, J.J. and Martin, D.P. 2005. Analysis of nighttime activity and daytime pain in patients with chronic back pain using a self-organizing map neural network. Journal of Clinical Monitoring and Computing. 19, 6 (2005), 411–414.
[20] Magnetometer Hookup Guide: https://learn.sparkfun.com/tutorials/mag3110-magnetometer-hookup-guide-?_ga=1.158885299.804409743.1473347658. Accessed: 2017-01-17.
[21] Makikawa, M., Shiozawa, N. and Okada, S. 2014. Fundamentals of Wearable Sensors for the Monitoring of Physical and Physiological Changes in Daily Life. Wearable Sensors: Fundamentals, Implementation and Applications. E. Sazonov and M. Neuman, eds. Elsevier. 517–541.
[22] Meijer, G.A.L., Westerterp, K.R., Verhoeven, F.M.H., Koper, H.B.M. and Hoor, F. ten 1991. Methods to Assess Physical Activity with Special Referrence to Motion Sensors and Accelerometers. Transactions on Biomedical Engineering. 38, 3 (1991), 221–229.
[23] Menz, H.B., Lord, S.R. and Fitzpatrick, R.C. 2003. Acceleration patterns of the head and pel v is when walking on le v el and irregular surfaces. Gait & posture. 18, 1 (2003), 35–46.
[24] Menz, H.B., Lord, S.R. and Fitzpatrick, R.C. 2003. Age-related differences in walking stability. Age and Ageing. 32, 2 (2003), 137–142.
[25] Mitchell, T., Madgwick, S. and Heap, I. 2012. Musical Interaction with Hand Posture and Orientation: A Toolbox of Gestural Control Mechanisms. Proceedings of the International Conference on New Interfaces for Musical Expression. May (2012), 21–23.
[26] Najafi, B., Aminian, K., Paraschiv-Ionescu, A., Loew, F., Büla, C.J. and Robert, P. 2003. Ambulatory system for human motion analysis using a kinematic sensor: Monitoring of daily physical activity in the elderly. IEEE Transactions on Biomedical Engineering. 50, 6 (2003), 711–723.
[27] Noury, N., Dittmar, A., Corroy, C., Baghai, R., Weber, J.L., Blanc, D., Klefstat, F., Blinovska, A., Vaysse, S. and Comet, B. 2004. VTAMN–a smart clothe for ambulatory remote monitoring of physiological parameters and activity. Conf Proc IEEE Eng Med Biol Soc (2004), 3266–3269.
[28] Park, J.-H., Kim, H.J., Kang, S.-J. and Kang, M. 2006. Validation of the AMP331 monitor for assessing energy expenditure of free-living physical activity. Res. Quart. Exerc. Sport. 77, (2006), A40.
[29] Sekine, M., Tamura, T., Togawa, T. and Fukui, Y. 2000. Classification of waist-acceleration signals in a continuous walking record. Medical Engineering and Physics. 22, 4 (2000), 285–291.
[30] Serafin, S., Trento, S., Grani, F., Perner-Wilson, H., Madgwick, S. and Mitchell, T. 2014. Controlling Physically Based Virtual Musical Instruments Using The Gloves. Proceedings of the International Conference on New Interfaces for Musical Expression. July (2014), 521–524.
[31] Sturman, D.J. and Zeltzer, D. 1994. A survey of glove-based input. Computer Graphics and Applications, IEEE. 14, 1 (1994), 30–39.
[32] Waltz, E. 2015. A WearableTurns Baseball Pitching Into a Science. IEEE Spectrum.
[33] Williamson, R. and Andrews, B.J. 2001. Detecting absolute human knee angle and angular velocity using accelerometers and rate gyroscopes. Medical & biological engineering & computing. 39, 3 (2001), 294–302.
[34] Yang, C.C. and Hsu, Y.L. 2010. A review of accelerometry-based wearable motion detectors for physical activity monitoring. Sensors. 10, 8 (2010), 7772–7788.
[35] Yang, C.C. and Hsu, Y.L. 2009. Development of a Wearable Motion Detector for Telemonitoring and Real-Time Identification of Physical Activity. TELEMEDICINE and e-HEALTH. (2009), 62–72.
[36] Zeagler, C., Starner, T., Hall, T. and Wong Sala, M. 2015. Meeting the Challenge: The Path Towards a Consumer Wearable Computer. Georgia Institute of Technology.
[37] Zhao, Y., Salunke, S., Leavitt, A., Curtin, K., Huynh, N. and Zeagler, C. 2016. E-Archery: Prototype Wearable for Analyzing Archery Release. Proceedings of the 2016 ACM International Joint Conference on Pervasive and Ubiquitous Computing Adjunct – UbiComp ’16. (2016), 908–913.
Active touch represents the exploratory action of touching.
Design Considerations for Active Touch
- Following good human factors and industrial design standards when creating physical interfaces will aid in a person’s ability to use active touch to interact with objects and controls. This is also true for interfaces on the surface of wearable devices.
- Certain shapes contain certain affordances. Concavities on top of buttons might lend themselves to a pushing type active touch investigation. Ridges on the circumference of cylinders might lend themselves to turning. Expenditures at an angle to a plane might afford a flick, or leverage. Dreyfus lays out shapes and sizes for controls in his book [7].
Accessibility Considerations Active Touch
- It is important to remember that each person has a different ability to feel, or sense tactile sensation. Thus interfaces should be designed with robust multisensory feedback. Where as one person might feel a click of a button through tactile means, others who cannot might require an audio cue or a visual cue to know that a selection has been made.
[1] Gibson, J. 1962. Observations on active touch. Psychological review. 69, 6 (1962), 477–491.
[2] Komor, N., Gilliland, S., Clawson, J., Manish, B., Garg, M., Zeagler, C. and Starner, T. 2009. Is It Gropable?–Assessing the Impact of Mobility on Textile Interfaces. International Symposium on Wearable Computers, ISWC (2009), 71–74.
[3] Lee, S. 2012. Buzzwear: Supporting multitasking with wearable tactile displays on the wrist.
[4] Loomis, J.M. and Lederman, S.J. 1986. Tactual Perception. Handbook of Perception and Human Performance.
[5] Loomis, J.M. and Lederman, S.J. 1984. What utility is there in distinguishing between active and passive touch? presentation at Psychonomic Society meeting (1984).
[6] Norman, D.A. 2013. The Design of Everyday Things.
[7] Tiley, A.R. and Henry Dreyfuss Associates 2001. The Measure Of Man And Woman: Human Factors In Design. John Wiley & Sons.
[8] Walters, K., Lee, S., Starner, T., Leibrandt, R. and Lawo, M. 2010. Touchfire: Towards a glove-mounted tactile display for rendering temperature readings for firefighters. Proceedings – International Symposium on Wearable Computers, ISWC. (2010).
[9] Watkins, S.M. and Dunne, L.E. 2015. Functional Clothing Design: From Sportswear to Spacesuits. Bloomsbury Publishing.
Average distance in two-point discrimination sensitivity test on body locations.
Design Considerations for Tangible / Tactile / Haptic Feedback (passive touch)
- When designing haptic displays for wearable devices, the sensitivity of the on-body location where the wearable device is placed very important.
- Vibrotactile displays should be programed to account for masking and vibrotactile adaptation.
Accessibility Considerations for Tangible / Tactile / Haptic Feedback (passive touch)
- Tangible/Haptic Feedback is an important part of a multimodal display system. Multimodal feedback is important; designers need to create wearable devices that can prompt users with a variety of different abilities. Vibration and haptic alerts can aid those with visual impairments when acoustic feedback is inappropriate.
- Vibration and haptic feedback have been seen to provide added benefit in rehabilitation of injuries (such as spinal injuries) where sensation has been degraded. Mobile Music Touch has shown that rehabilitation with the vibrating piano gloves not only taught participants to play piano, but also improved their sensation and dexterity [28].
[1] “AB-004 : UNDERSTANDING ERM VIBRATION MOTOR CHARACTERISTICS.” 2017. Accessed February 6. https://www.precisionmicrodrives.com/application-notes/ab-004-understanding-erm-vibration-motor-characteristics.
[2] “AB-020 : UNDERSTANDING LINEAR RESONANT ACTUATOR CHARACTERISTICS.” 2017. Accessed July 2. https://www.precisionmicrodrives.com/application-notes/ab-020-understanding-linear-resonant-actuator-characteristics.
[3] Benedetti, F. 1988. “Localization of Tactile Stimuli and Body Parts in Space: Two Dissociated Perceptual Experiences Revealed by a Lack of Constancy in the Presence of Position Sense and Motor Activity.”
Journal of Experimental Psychology. Human Perception and Performance 14 (1): 69–76. doi:10.1037/0096-1523.14.1.69.
[4] Bensmaia, S. J., Y.Y. Leung, S.S. Hsiao, and K.O. Johnson. 2005. “Vibratory Adaptation of Cutaneous Mechanoreceptive Afferents.”
Journal of Neurophysiology 94 (5): 3023–36. doi:10.1152/jn.00002.2005.
[5] Brown, Lorna M., Stephen a. Brewster, and Helen C. Purchase. 2006. “Multidimensional Tactons for Non-Visual Information Presentation in Mobile Devices.”
Proceedings of the 8th Conference on Human-Computer Interaction with Mobile Devices and Services – MobileHCI ’06 159 (September): 231–38. doi:10.1145/1152215.1152265.
[6] Capraro, A.J., R.T. Verrillo, and J.J. Zwislocki. 1979. “Psychophysical Evidence for a Triplex System of Cutaneous Mechanoreception.”
Sensory Processes 3: 340–52.
[7] Cholewiak, R W, and a a Collins. 1995. “Vibrotactile Pattern Discrimination and Communality at Several Body Sites.”
Perception & Psychophysics 57 (6): 724–37. doi:10.3758/BF03213276.
[8] Craig, J C, and P M Evans. 1987. “Vibrotactile Masking and the Persistence of Tactual Features.”
Perception & Psychophysics 42 (4): 309–17. doi:10.3758/BF03203085.
[9] Craig, James C. 2002. “Identification of Scanned and Static Tactile Patterns.”
Perception & Psychophysics 64 (1): 107–20. doi:10.3758/BF03194560.
[10] Craig, James C. 1983. “Some Factors Affecting Tactile Pattern Recognition.”
International Journal of Neuroscience 19 (1–4): 47–57.
[11] Craig, James C. 1985. “Tactile Pattern Perception and Its Perturbations.”
The Journal of the Acoustical Society of America 77 (1): 238. doi:10.1121/1.392265.
[12] Erp, Jan B. F. van. 2002. “Guidelines for the Use of Vibro-Tactile Displays in Human Computer Interaction.”
Proceedings of Eurohaptics, 18–22. doi:10.1016/j.ajodo.2008.04.017.
[13] Erp, Jan Van. 2005. “Presenting Directions with a Vibrotactile Torso Display.”
Ergonomics 48 (3): 302–13. doi:10.1080/0014013042000327670.
[14] Feygin, D., M. Keehner, and R. Tendick. 2002. “Haptic Guidance: Experimental Evaluation of a Haptic Training\nmethod for a Perceptual Motor Skill.”
Proceedings 10th Symposium on Haptic Interfaces for Virtual Environment and Teleoperator Systems. HAPTICS 2002, no. March: 40–47. doi:10.1109/HAPTIC.2002.998939.
[15] Gallace, A., H.Z. Tan, and C. Spence. 2005. “Tactile Change Detection.”
First Joint Eurohaptics Conference and Symposium on Haptic Interfaces for Virtual Environment and Teleoperator Systems. World Haptics Conference, 0–4. doi:10.1109/WHC.2005.122.
[16] Gallace, Alberto, Malika Auvray, Hong Z. Tan, and Charles Spence. 2006. “When Visual Transients Impair Tactile Change Detection: A Novel Case of Crossmodal Change Blindness?”
Neuroscience Letters 398 (3): 280–85. doi:10.1016/j.neulet.2006.01.009.
[17] Gallace, Alberto, Hong Z. Tan, and Charles Spence. 2007. “The Body Surface as a Communication System: The State of the Art after 50 Years.”
Presence: Teleoperators and Virtual Environments 16 (6): 655–76. doi:10.1162/pres.16.6.655.
[18] Geldard, F.A., and C.E. Sherrick. 1972. “The Cutaneous ‘rabbit’: A Perceptual Illusion.”
Science 178: 178–79.
[19] Gescheider, G.A., R. T. Verrillo, A. J. Capraro, and R. D. Hamer. 1977. “Enhancement of Vibrotactile Sensation Magnitude and Predictions from the Duplex Model of Mechanoreception.”
Sensory Processes 1 (3): 187–203.
[20] Gescheider, George A., Stanley J. Bolanowski, Ronald T. Verrillo, Dean J. Arpajian, and Timothy F. Ryan. 1990. “Vibrotactile Intensity Discrimination Measured by Three Methods.”
The Journal of the Acoustical Society of America 87 (1): 330–38. doi:10.1121/1.399300.
[21] Gibson, JJ. 1962. “Observations on Active Touch.”
Psychological Review 69 (6): 477–91. doi:10.1037/h0046962.
[22] Hahn, J.F. 1966. “Vibrotactile Adaptation and Recovery Measured by Two Methods.”
Journal of Experimental Psychology 71 (5): 655–58.
[23] Huang, Kevin, Thad Starner, Ellen Do, Gil Weinberg, Georgia Tech, Daniel Kohlsdorf, Claas Ahlrichs, and Ruediger Leibrandt. 2010. “Mobile Music Touch : Mobile Tactile Stimulation For Passive Learning,” 791–800.
[24] Kirman, Jacob H. 1974. “Tactile Apparent Movement: The Effects of Number of Stimulators.”
Journal of Experimental Psychology 103 (6): 1175–80. doi:10.3758/BF03205819.
[25] Kruijff, Ernst, Dieter Schmalstieg, and Steffi Beckhaus. 2006. “Using Neuromuscular Electrical Stimulation for Pseudo-Haptic Feedback.”
Proceedings of the ACM Symposium on Virtual Reality Software and Technology – VRST ’06, 316. doi:10.1145/1180495.1180558.
[26] Lee, Seungyon. 2012. “Buzzwear: Supporting Multitasking with Wearable Tactile Displays on the Wrist.”
Dissertation Abstracts International: Section B: The Sciences and Engineering. http://gateway.proquest.com/openurl?url_ver=Z39.88-2004&rft_val_fmt=info:ofi/fmt:kev:mtx:dissertation&res_dat=xri:pqdiss&rft_dat=xri:pqdiss:3455269%5Cnhttp://ovidsp.ovid.com/ovidweb.cgi?T=JS&PAGE=reference&D=psyc7&NEWS=N&AN=2012-99020-016.
[27] Mancini, Flavia, Armando Bauleo, Jonathan Cole, Fausta Lui, Carlo A. Porro, Patrick Haggard, and Gian Domenico Iannetti. 2014. “Whole-Body Mapping of Spatial Acuity for Pain and Touch.”
Annals of Neurology 75 (6): 917–24. doi:10.1002/ana.24179.
[28] Markow, T, N Ramakrishnan, K Huang, T Starner, C Schooler, A Tarun, United States, and United States. n.d. “Mobile Music Touch : Vibration Stimulus in Hand Rehabilitation.”
[29] Nolan, M F. 1982. “Two-Point Discrimination Assessment in the Upper Limb in Young-Adult Men and Women.”
Physical Therapy 62 (7): 965–69. %3CGo.
[30] Oakley, Ian, Yeongmi Kim, Junhun Lee, and Jeha Ryu. 2006. “Determining the Feasibility of Forearm Mounted Vibrotactile Displays.”
Proceedings – IEEE Virtual Reality 2006: 74. doi:10.1109/VR.2006.49.
[31] Pasquero, J. 2006. “Survey on Communication through Touch.”
Center for Intelligent Machines-McGill University, Tech. Rep. TR-CIM 6 (August): 1–28. http://scholar.google.com/scholar?hl=en&btnG=Search&q=intitle:Survey+on+Communication+through+Touch#0.
[32] Schiffman, Harvey Richard. 2001. “The Skin Senses.” In
Sensation and Perception, Fifth, 412–49. John Wiley & Sons, Inc.
[33] Seim, Caitlyn, John Chandler, Kayla DesPortes, Siddharth Dhingra, Miru Park, and Thad Starner. 2014. “Passive Haptic Learning of Braille Typing.”
Proceedings of the 2014 ACM International Symposium on Wearable Computers – ISWC ’14, 111–18. doi:10.1145/2634317.2634330.
[34] Seim, Caitlyn E, David Quigley, and Thad E Starner. 2014. “Passive Haptic Learning of Typing Skills Facilitated by Wearable Computers.”
Proceedings of the Extended Abstracts of the 32nd Annual ACM Conference on Human Factors in Computing Systems – CHI EA ’14, 2203–8. doi:10.1145/2559206.2581329.
[35] Seim, Caitlyn, Tanya Estes, and Thad Starner. 2015. “Towards Passive Haptic Learning of Piano Songs.”
IEEE World Haptics Conference, WHC 2015, 445–50. doi:10.1109/WHC.2015.7177752.
[36] Seim, Caitlyn, James Hallam, Shashank Raghu, Tri-an Le, Greg Bishop, and Thad Starner. 2015. “Perception in Hand-Worn Haptics : Placement , Simultaneous Stimuli , and Vibration Motor Comparisons.” https://smartech.gatech.edu/handle/1853/55952.
[37] Sherrick, C E, R W Cholewiak, and A A Collins. 1990. “The Localization of Low- and High-Frequency Vibrotactile Stimuli.”
J. Acoust. Soc. Am. 88 (1): 169–79. doi:10.1121/1.399937.
[38] Sherrick, Carl E. 1968. “Studies of Apparent Tactual Movement.”
The Skin Senses, 331–44.
[39] Toney, A, L Dunne, B.H. Thomas, and S.P. Ashdown. 2003. “A Shoulder Pad Insert Vibrotactile Display.”
Seventh IEEE International Symposium on Wearable Computers, 2003. Proceedings., 35–44. doi:10.1109/ISWC.2003.1241391.
[40] Verillo, R. T., and G. A. Gescheider. 1977. “Effect of Prior Stimulation on Vibrotactile Thresholds.”
Sensory Processes, no. 1: 292–300.
[41] Verrillo, R.T. 1985. “Psychophysics of Vibrotactile Stimulation.”
The Journal of the Acoustical Society of America 77 (1): 225–32. doi:10.1121/1.392263.
[42] Verrillo, R T, G A Gescheider, B G Calman, and C L Van Doren. 1983. “Vibrotactile Masking: Effects of One- and Two-Site Stimulation.”
Perception & Psychophysics 33 (4): 379–87. doi:10.3758/BF03205886.
[43] Verrillo, Ronald T., and George a. Gescheider. 1975. “Enhancement and Summation in the Perception of Two Successive Vibrotactile Stimuli.”
Perception & Psychophysics 18 (2): 128–36. doi:10.3758/BF03204100.
[44] Weber EH. n.d.
De Subtilitate Tactus. Edited by Ross HE and Murray DJ. 2nd ed.
[45] Weinstein S. 1968. “Intensive and Extensive Aspects of Tactile Sensitivity as a Function of Body Part, Sex, and Laterality.” In
The Skin Senses, edited by Kenshalo D.R., 195–222. Springfield, IL.
This body map shows where to place wearable technology (which adds heat through operation and added bulk and material). This map focuses on a devices tendency to raise overall body temperature; however, tissue on the body can burn at any location is exposed to enough heat over time.
Design Considerations for Thermal Tolerances
- In a hot environment, a wearable “should be non-conductive (so the from the environment or heat produced by the wearable is not conducted to the body).” [27]
- In a hot environment, a wearable should “allow air to circulate as freely as possible over the skin surface (to aid convective cooling and evaporation of sweat).” [27]
- “Allow freedom of movement (to prevent any extra physical effort that would increase metabolism).” [27]
- In a hot environment, a wearable “should provide minimum coverage or maximum ventilation for the body core.” [27]
- In a cold environment, the heat produced by the wearable could be directed towards the body for extra warmth. [27]
- Do not allow devices to exceed 105°F at any time when next to the skin.
- A temperature of 111.2°F for 7 hours next to the skin can cause a second or third degree burn.
Accessibility Considerations for Thermal Tolerances
- Those with sensory disabilities could be highly susceptible to burns from wearable devices, and as such temperature sensors should be placed in wearable devices with cut off mechanisms.
- Multimodal displays including lights and sound should be used to warn of overheating. Again if a person cannot feel a localized heat source at the body location where the wearable is placed, even lower temperatures over longer time periods can cause significant burns.
[1] Charkoudian, N. 2003. Skin blood flow in adult human thermoregulation: how it works, when it does not, and why. Mayo Clinic proceedings. 78, 5 (2003), 603–12.
[2] Chato, J.C. 1980. Heat transfer to blood vessels. Journal of biomechanical engineering. 102, 2 (1980), 110–118.
[3] de Dear, R.J., Arens, E., Hui, Z. and Oguro, M. 1997. Convective and radiative heat transfer coefficients for individual human body segments. International journal of biometeorology. 40, 3 (1997), 141–156.
[4] Defrin, R., Schachal-shiffer, M., Hadgadg, M. and Peretz, C. 2006. Quantitative Somatosensory Testing of Warm and Heat-Pain Thresholds: The Effect of Body Region and Testing Method. The Clinical Journal of Pain. 22, 2 (2006), 130–136.
[5] Fire Dynamics: https://www.nist.gov/%3Cfront%3E/fire-dynamics. Accessed: 2017-01-18.
[6] Gemperle, F., Kasabach, C., Stivoric, J., Bauer, M. and Martin, R. 1998. Design for wearability. Digest of Papers. Second International Symposium on Wearable Computers (1998).
[7] Greene, L.C. and Hardy, J.D. 1962. Adaptation of Thermal Pain in the Skin. Journal of Applied Physiology. 17, 4 (1962), 693–696.
[8] Huizenga, C., Hui, Z. and Arens, E. 2001. A model of human physiology and comfort for assessing complex thermal environments. Building and Environment. 36, 6 (2001), 691–699.
[9] ISO 13732-1:2006(en) Ergonomics of the thermal environment — Methods for the assessment of human responses to contact with surfaces — Part 1: Hot surfaces: 2006. https://www.iso.org/obp/ui/#iso:std:iso:13732:-1:ed-1:v1:en. Accessed: 2017-01-18.
[10] Leoni, N., Amon, C.H. and Member, S. 2000. Bayesian Surrogates for Integrating Numerical , Analytical , and Experimental Data : Application to Inverse Heat Transfer in Wearable Computers. 23, 1 (2000), 23–32.
[11] Lloyd-Smith, D.L. and Mendelssohn, K. 1948. Tolerance Limits to Radiant Heat. British Medical Journal. 1, 4559 (1948), 975–978.
[12] Melikov, A.K., Cermak, R. and Majer, M. 2002. Personalized ventilation: Evaluation of different air terminal devices. Energy and Buildings. 34, 8 (2002), 829–836.
[13] Moritz, A.R. and Henriques, F.C. 1947. Studies of thermal injury: the relative importance of time and surface temperature in the causation of cutaneous burns. American Journal of Pathology. 23, (1947), 695–720.
[14] Murakami, S., Kato, S. and Zeng, J. 2000. Combined simulation of airflow, radiation and moisture transport for heat release from a human body. Building and Environment. 35, 6 (2000), 489–500.
[15] Peenes, H.H. 1948. Analysis of Tissue and Arterial Blood Temperatures in the Resting Human Forearm. Journal of Applied Physiology. 1, 2 (1948), 93–122.
[16] Ring, J.W. and de Dear, R.J. 1991. Temperature Transients: A Model for Heat Diffusion through the Skin, Thermoreceptor Response and Thermal Sensation. Indoor Air. 1, 4 (1991), 448–456.
[17] Sallam, H. and Jenkins, M.D. 2000. Modular Wearable Computer. 6,157,533. 2000.
[18] Song, W.J., Weinbaum, S., Jiji, L.M. and Lemons, D. 1988. A combined macro and microvascular model for whole limb heat transfer. Journal of Biomechanical Engineering. 110, 4 (1988), 259–268.
[19] Sørensen, D.N. and Voigt, L.K. 2003. Modelling flow and heat transfer around a seated human body by computational fluid dynamics. Building and Environment. 38, 6 (2003), 753–762.
[20] Starner, T. 1996. Human-powered wearable computing. IBM Systems Journal. 35, 3.4 (1996), 618–629.
[21] Starner, T. 2001. The Challenges of Wearable computing: Part 1. IEEE Micro. 21, (2001), 44–52.
[22] Starner, T. 2001. The Challenges of Wearable Computing: Part 2. IEEE Micro. 21, (2001), 54–67.
[23] Starner, T. 2015. Wearable Computing: Meeting the Challenge. Fundamentals of Wearable Computers and Augmented Reality, Second Edition. W. Barfield, ed. CRC Press.
[24] Stoll, A.M., Chianta, M.A. and Piergallini, J.R. 1979. Thermal conduction effects in human skin. Aviation, Space, and Environmental Medicine. 50, 8 (1979), 778–787.
[25] Temperature / Time / Burn Chart: https://www.armstronginternational.com/sites/default/files/resources/documents/ay-699.pdf. Accessed: 2017-01-18.
[26] Ungar, E. and Stroud, K. 2010. A New Approach to Defining Human Touch Temperature Standards. 40th International Conference on Environmental Systems (ICES). (2010), 10.
[27] Watkins, S.M. 1995. Clothing: The Portable Environment. Iowa State Press.
[28] Zeagler, C., Starner, T., Hall, T. and Wong Sala, M. 2015. Meeting the Challenge: The Path Towards a Consumer Wearable Computer. Georgia Institute of Technology.
An Electrocardiogram (ECG) monitor will usually have to be located on the chest, or arms depending on the preciseness of measurement needed. Whereas heart rate can be determined by a pulse oximeter or photoplethysmography (PPG) placed where blood vessels are close to the surface of the skin.
[1] Asada, H.H., Shaltis, P., Reisner, A., Rhee, S. and Hutchinson, R.C. 2003. Mobile Monitoring with Wearable Photoplethysmographic Biosensors. IEEE Engineering in Medicine and Biology Magazine. 22, 3 (2003), 28–40.
[2] Howell, T.A., Chao, D., Thomas, D., Day, R.G. and Tong, P.P. 2010. Eyeglasses With a Heart Rate. US 7,677,723 B2. 2010.
[3] Lu, S., Zhao, H., Ju, K., Shin, K., Lee, M., Shelley, K. and Chon, K.H. 2008. Can photoplethysmography variability serve as an alternative approach to obtain heart rate variability information? Journal of Clinical Monitoring and Computing. 22, 1 (2008), 23–29.
[4] Pantelopoulos, A. and Bourbakis, N. 2008. A survey on wearable biosensor systems for health monitoring. Proceedings of the 30th Annual International Conference of the IEEE Engineering in Medicine and Biology Society, EMBS’08 – “Personalized Healthcare through Technology.” (2008), 4887–4890.
[5] Pantelopoulos, A. and Bourbakis, N.G. 2010. A survey on wearable sensor-based systems for health monitoring and prognosis. IEEE Transactions on Systems, Man and Cybernetics Part C: Applications and Reviews. 40, 1 (2010), 1–12.
[6] Poh, M., Division, H., Sciences, H. and Kim, K. 2012. Cardiovascular Monitoring Using Earphones and a Mobile Device. IEEE Pervasive Computing. 4, 11 (2012), 18–26.
[7] Schäfer, A. and Vagedes, J. 2013. How accurate is pulse rate variability as an estimate of heart rate variability?: A review on studies comparing photoplethysmographic technology with an electrocardiogram. International Journal of Cardiology. 166, 1 (2013), 15–29.
[8] Shelley, K.H. 2007. Photoplethysmography: Beyond the calculation of arterial oxygen saturation and heart rate. Anesthesia and Analgesia. 105, SUPPL. 6 (2007), 31–36.
[9] Sterling, G.J., Mazeika, J. and Rissman, W. 2009. HEART MONITORING BODY PATCH AND SYSTEM. 20090062670 A1. 2009.
[10] Sweeny, J.S. 1977. BLOOD PULSE SENSOR AND READOUT. 4063551. 1977.
[11] Tamura, T., Maeda, Y., Sekine, M. and Yoshida, M. 2014. Wearable Photoplethysmographic Sensors—Past and Present. Electronics. 3, 2 (2014), 282–302.
[12] Watkins, S.M. and Dunne, L.E. 2015. Functional Clothing Design: From Sportswear to Spacesuits. Bloomsbury Publishing.
[13] Yang, G.Z., Lo, B.P.L. and Wang, L. 2010. HEART RATE MEASUREMENT. 20100113948 A1. 2010.
[14] Yousefi, R., Nourani, M., Ostadabbas, S. and Panahi, I. 2014. A motion-tolerant adaptive algorithm for wearable photoplethysmographic biosensors. IEEE Journal of Biomedical and Health Informatics. 18, 2 (2014), 670–681.
Photoplethysmography (PPG) sensors can also capture blood pressure when placed correctly on the body. PPG sensors are easier to wear all day than traditional blood pressure sensors.
[1] Makikawa, M., Shiozawa, N. and Okada, S. 2014. Fundamentals of Wearable Sensors for the Monitoring of Physical and Physiological Changes in Daily Life. Wearable Sensors: Fundamentals, Implementation and Applications. E. Sazonov and M. Neuman, eds. Elsevier. 517–541.
[2] McCombie, D. and Reisner, A. 2010. Wearable pulse wave velocity blood pressure sensor and methods of calibration thereof. US Patent …. 2, 12 (2010).
[3] Pantelopoulos, A. and Bourbakis, N. 2008. A survey on wearable biosensor systems for health monitoring. Proceedings of the 30th Annual International Conference of the IEEE Engineering in Medicine and Biology Society, EMBS’08 – “Personalized Healthcare through Technology.” (2008), 4887–4890.
[4] Pantelopoulos, A. and Bourbakis, N.G. 2010. A survey on wearable sensor-based systems for health monitoring and prognosis. IEEE Transactions on Systems, Man and Cybernetics Part C: Applications and Reviews. 40, 1 (2010), 1–12.
[5] Watkins, S.M. and Dunne, L.E. 2015. Functional Clothing Design: From Sportswear to Spacesuits. Bloomsbury Publishing.
Thermistors can also be used to measure temperature (can be worn in the nostril or in the rectum). A thermophile made of many thermocouples can be worn in the ear. Thermocouples can also be used in smart watches and fitness bands to estimate body temperature.
[1] Makikawa, M., Shiozawa, N. and Okada, S. 2014. Fundamentals of Wearable Sensors for the Monitoring of Physical and Physiological Changes in Daily Life. Wearable Sensors: Fundamentals, Implementation and Applications. E. Sazonov and M. Neuman, eds. Elsevier. 517–541.
[2] Pantelopoulos, A. and Bourbakis, N. 2008. A survey on wearable biosensor systems for health monitoring. Proceedings of the 30th Annual International Conference of the IEEE Engineering in Medicine and Biology Society, EMBS’08 – “Personalized Healthcare through Technology.” (2008), 4887–4890.
[3] Pantelopoulos, A. and Bourbakis, N.G. 2010. A survey on wearable sensor-based systems for health monitoring and prognosis. IEEE Transactions on Systems, Man and Cybernetics Part C: Applications and Reviews. 40, 1 (2010), 1–12.
[4] Watkins, S.M. and Dunne, L.E. 2015. Functional Clothing Design: From Sportswear to Spacesuits. Bloomsbury Publishing.
Most respiration monitors actually measure chest cavity expansion through a piezoelectric / piezoresistive sensor, or through electrical resistance change across a knitted conductive textile. A thermistor can be placed inside the nostril to detect breathing patterns.
[1] Chiarugi, F., Karatzanis, I., Zacharioudakis, G., Meriggi, P., Rizzo, F., Stratakis, M., Louloudakis, S., Biniaris, C., Valentini, M., Di Rienzo, M. and Parati, G. 2008. Measurement of heart rate and respiratory rate using a textile-based wearable device in heart failure patients. Computers in Cardiology. 35, (2008), 901–904.
[2] Choi, S. and Jiang, Z. 2006. A novel wearable sensor device with conductive fabric and PVDF film for monitoring cardiorespiratory signals. Sensors and Actuators, A: Physical. 128, 2 (2006), 317–326.
[3] Makikawa, M., Shiozawa, N. and Okada, S. 2014. Fundamentals of Wearable Sensors for the Monitoring of Physical and Physiological Changes in Daily Life. Wearable Sensors: Fundamentals, Implementation and Applications. E. Sazonov and M. Neuman, eds. Elsevier. 517–541.
[4] Pantelopoulos, A. and Bourbakis, N. 2008. A survey on wearable biosensor systems for health monitoring. Proceedings of the 30th Annual International Conference of the IEEE Engineering in Medicine and Biology Society, EMBS’08 – “Personalized Healthcare through Technology.” (2008), 4887–4890.
[5] Pantelopoulos, A. and Bourbakis, N.G. 2010. A survey on wearable sensor-based systems for health monitoring and prognosis. IEEE Transactions on Systems, Man and Cybernetics Part C: Applications and Reviews. 40, 1 (2010), 1–12.
[6] Rovira, C., Coyle, S., Corcoran, B., Diamond, D., Stroiescu, F. and Daly, K. 2011. Integration of textile-based sensors and Shimmer for breathing rate and volume measurement. International Conference on Pervasive Computing Technologies for Healthcare. m, (2011), 238–241.
[7] Watkins, S.M. and Dunne, L.E. 2015. Functional Clothing Design: From Sportswear to Spacesuits. Bloomsbury Publishing.
Most blood glucose monitors are worn at the waist, but advances might soon see contact lens glucose monitors.
[1] Badugu, R., Lakowicz, J.R. and Geddes, C.D. 2005. A glucose-sensing contact lens: From bench top to patient. Current Opinion in Biotechnology. 16, 1 SPEC. ISS. (2005), 100–107.
[2] Badugu, R., Lakowicz, J.R. and Geddes, C.D. 2003. A Glucose Sensing Contact Lens: A Non-Invasive Technique for Continuous Physiological Glucose Monitoring. Journal of Fluorescence. 13, 5 (2003), 371–374.
[3] Mastrototaro, J.J. 2000. The MiniMed continuous glucose monitoring System. Diabetes Technology and Therapeutics. 2, SUPPL. 1 (2000), S13–S18.
[4] Mohanty, S.P. and Kougianos, E. 2006. Biosensors : A Tutorial Review. IEEE Potentials. 25, 2 (2006), 35–40.
[5] Pantelopoulos, A. and Bourbakis, N. 2008. A survey on wearable biosensor systems for health monitoring. Proceedings of the 30th Annual International Conference of the IEEE Engineering in Medicine and Biology Society, EMBS’08 – “Personalized Healthcare through Technology.” (2008), 4887–4890.
[6] Pantelopoulos, A. and Bourbakis, N.G. 2010. A survey on wearable sensor-based systems for health monitoring and prognosis. IEEE Transactions on Systems, Man and Cybernetics Part C: Applications and Reviews. 40, 1 (2010), 1–12.
[7] Rashidi, P. and Mihailidis, A. 2013. A survey on ambient-assisted living tools for older adults. IEEE Journal of Biomedical and Health Informatics. 17, 3 (2013), 579–590.
[8] Watkins, S.M. and Dunne, L.E. 2015. Functional Clothing Design: From Sportswear to Spacesuits. Bloomsbury Publishing.
[9] Yao, H., Shum, A.J., Cowan, M., Lähdesmäki, I. and Parviz, B.A. 2011. A contact lens with embedded sensor for monitoring tear glucose level. Biosensors and Bioelectronics. 26, 7 (2011), 3290–3296.
[10] Badugu, R., Lakowicz, J.R. and Geddes, C.D. 2004. Ophthalmic glucose sensing: A novel monosaccharide sensing disposable and colorless contact lens.
Analyst. 129, 6 (2004), 516–521. DOI:https://doi.org/10.1039/b314463c.
[11] Badugu, R., Reece, E.A. and Lakowicz, J.R. 2018. Glucose-sensitive silicone hydrogel contact lens toward tear glucose monitoring.
Journal of Biomedical Optics. 23, 05 (2018), 1. DOI:https://doi.org/10.1117/1.jbo.23.5.057005.
Galvanic Skin Response GSR data can give quite a number of insights including stress, hydration, and of course perspiration. These measurements can be taken from anywhere on the skin once calibrated to a location.
[1] Cole, P.J., Lemura, L.M., Klinger, T.A., Strohecker, K. and McConnell, T.R. 2004. Measuring energy expenditure in cardiac patients using the Body Media ( TM ) Arm … Sports Medicine. (2004).
[2] Gao, W., Emaminejad, S., Nyein, H.Y.Y., Challa, S., Chen, K., Peck, A., Fahad, H.M., Ota, H., Shiraki, H., Kiriya, D., Lien, D.-H., Brooks, G.A., Davis, R.W. and Javey, A. 2016. Fully integrated wearable sensor arrays for multiplexed in situ perspiration analysis. Nature. 529, 7587 (2016), 509–514.
[3] Hernandez, J., Riobo, I., Rozga, A., Abowd, G.D. and Picard, R.W. 2014. Using electrodermal activity to recognize ease of engagement in children during social interactions. Proceedings of the 2014 ACM International Joint Conference on Pervasive and Ubiquitous Computing – UbiComp ’14 Adjunct. (2014), 307–317.
[4] Huang, X., Liu, Y., Cheng, H., Shin, W.J., Fan, J.A., Liu, Z., Lu, C.J., Kong, G.W., Chen, K., Patnaik, D., Lee, S.H., Hage-Ali, S., Huang, Y. and Rogers, J.A. 2014. Materials and designs for wireless epidermal sensors of hydration and strain. Advanced Functional Materials. 24, 25 (2014), 3846–3854.
[5] Kientz, J.A., Goodwin, M.S., Hayes, G.R. and Abowd, G.D. 2013. Interactive Technologies for Autism. Morgan & Claypool Publishers.
[6] Marchand, G., Bourgerette, A., Antonakios, M., Colletta, Y., David, N., Vinet, F. and Gallis, C. 2010. Development of a hydration sensor integrated on fabric. Proceedings of the 6th International Workshop on Wearable, Micro, and Nano Technologies for Personalized Health: “Facing Future Healthcare Needs”, pHealth 2009. (2010), 37–40.
[7] Matzeu, G., Florea, L. and Diamond, D. 2015. Advances in wearable chemical sensor design for monitoring biological fluids. Sensors and Actuators, B: Chemical. 211, (2015), 403–418.
[8] Pantelopoulos, A. and Bourbakis, N. 2008. A survey on wearable biosensor systems for health monitoring. Proceedings of the 30th Annual International Conference of the IEEE Engineering in Medicine and Biology Society, EMBS’08 – “Personalized Healthcare through Technology.” (2008), 4887–4890.
[9] Pantelopoulos, A. and Bourbakis, N.G. 2010. A survey on wearable sensor-based systems for health monitoring and prognosis. IEEE Transactions on Systems, Man and Cybernetics Part C: Applications and Reviews. 40, 1 (2010), 1–12.
[10] Up 3 by Jawbone: https://jawbone.com/fitness-tracker/up3. Accessed: 2017-01-31.
[11] Watkins, S.M. and Dunne, L.E. 2015. Functional Clothing Design: From Sportswear to Spacesuits. Bloomsbury Publishing.
[12] Windmiller, J.R. and Wang, J. 2013. Wearable Electrochemical Sensors and Biosensors: A Review. Electroanalysis. 25, 1 (2013), 29–46.
[13] Zeagler, C., Starner, T., Hall, T. and Wong Sala, M. 2015. Meeting the Challenge: The Path Towards a Consumer Wearable Computer. Georgia Institute of Technology.
Design Considerations for Biometric Sensing
- Heart Rate / Heart Movement ECG sensors tend to be located on the chest near the heart. The more precise measurement needed the more body area these electrode-based sensors will cover.
- Stimulation/Perspiration/Hydration sensors can be placed anywhere on the body with correct calibration, and thus should come secondary in body placement to other sensors packaged with them. These sensors need direct contact with the skin.
- Blood Pressure sensors and PPG Heart Rate sensors should be placed where blood vessels are close to the surface of the skin. These sensors also need direct contact with the skin.
- Blood Glucose sensors that have a subdermal port tend to be located at the waist (perhaps for ease of supporting the external electronics on the waistband). Other types of Blood Glucose monitors are located at the source of bodily fluid they are utilizing for sensing.
- Respiration monitor generally strap around the chest and measure chest volume change (they tend to be paired with a simple ECG to collect heart rate).
- EEG Sensors have to be placed on the scalp/forehead to measure brain activity.
Accessibility Considerations for Biometric Sensing
- Designing wireless wearable technology for collecting important biometric data might be one of the best ways to allow those who need to have constant monitoring an accessible and free lifestyle.
- New glucose monitor designs that do not require a subdermal port are less invasive, but might not yet collect data with the desired accuracy and reliability needed in some cases.
- EEG sensors that are mobile and wireless offer up great potential in accessibility, especially for “locked in” patients. These sensors might not be accurate enough yet for medical use.
- Choices in body location of biometric sensing for impaired individuals need to both work for the biosensor to collect data and for the mobility/reachability of the individual.
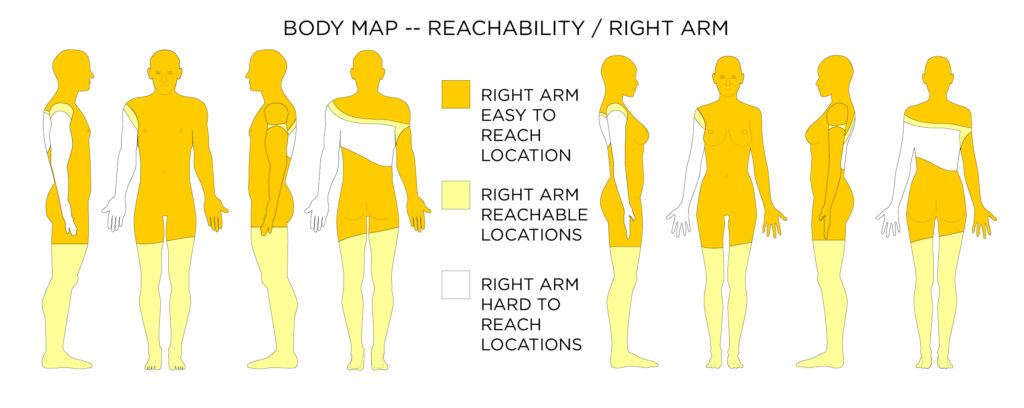
When it comes to reach-ability there are easy to reach locations (where your hand can reach without any body movement), reachable locations (where you can move a part of your body to your hand to be able to reach it), and hard to reach locations (such as your center back).
[1] Watkins, S.M. and Dunne, L.E. 2015. Functional Clothing Design: From Sportswear to Spacesuits. Bloomsbury Publishing.
Design Considerations for Reach-ability
- Wearable Devices should be placed in easy to reach on-body locations, especially for interfaces, but also for donning and doffing.
Accessibility Considerations Reach-ability
- Reachability is very personal as people have different physical abilities with respect to body movement.
- It is best to design a wearable device that does not cater to a dominant body side (right / left) and also is easy to reach with the hand’s extension.
- Some people who spend most of their time in a seated position might be able to reach their upper thighs to their knees more readily. It might be better however to design wearable devices useful to everyone in the same way.
Average reaction time to visible feedback
Design Considerations for Visible Feedback
- When designing a wearable device with a display or visual signal it is important that the device be placed on a part of the body where the display can be seen, and also a place on the body where it will be noticed easily.
Accessibility Considerations for Visible Feedback
- Visual displays should be accompanied by non-visual signals for those with visual impairments.
- The visibility of on-body locations might change from person to person depending on their mobility and means of mobility. Wheelchairs or other mobility devices might occlude some on-body locations, which would otherwise be acceptable for a visual display.
[1] Harrison, C., Benko, H. and Wilson, A.D. 2011. OmniTouch. Proceedings of the 24th annual ACM symposium on User interface software and technology – UIST ’11. (2011), 441.
[2] Harrison, C., Lim, B.Y., Shick, A. and Hudson, S.E. 2009. Where to Locate Wearable Displays? Reaction Time Performance of Visual Alerts from Tip to Toe. Proceedings of the 27th Annual SIGCHI Conference on Human Factors in Computing Systems. (2009), 941–944.
[3] Harrison, C., Ramamurthy, S. and Hudson, S.E. 2012. On-body interaction: armed and dangerous. Proceedings of TEI 2012. (2012), 69–76.
This body map shows the areas on the body where a network antenna (to communicate to the fixed off-body wireless frequencies) could be placed to have the least chance of signal interference by the mass of the body.
Design Considerations for Networking
- Antennas for wearable devices should be placed on the periphery of the body to have the best chance of having an unobstructed (by the body) connection to the fixed off-body network. This could mean the outer arms, shoulders or the head. Because of the strength and abundance of fixed off-body wireless network signal, this is not as much of a problem as it would have been in 2001.
- Body area Networked devices using low powered wireless connections between devices on the body should also try to avoid obstruction by the body between devices. For example if one device on the front torso needs to wirelessly communicate via low powered signal to a device on the back a third relay might be needed on the side of the body.
- All body mass compositions are unique. Outside of the general guidelines, wearable systems using wireless communication should be tested thoroughly on a variety of people, and in a variety of settings.
Accessibility Considerations for Networking
- Health monitors or wearable sensing devices use Body Area Networks. Some people might have many different monitors all using different frequencies. It is important when designing a new device that it does not interfere with wearable health devices such as heart monitors or pace makers. Its also important that it does not interfere with wireless hearing aids and other assistive devices. Adding a new signal to a series of signals requires some standards research and testing.
[1] Hall, S.P. and Hao, Y. 2006. Antennas and propagation for body centerric wireless communication. Proceedings EuCAP 2006 (Nice, France, 2006).
[2] Hanson, M.A., Powell, H.C., Barth, A.T., Ringgenberg, K., Calhoun, B.H., Aylor, J.H. and Lach, J. 2009. Body Area Sensor Networks: Challenges and Opportunities. Computer. 42, 1 (2009), 58–65.
[3] Movassaghi, S., Abolhasan, M., Lipman, J., Smith, D. and Jamalipour, A. 2014. Wireless Body Area Networks: A Survey. Ieee Communications Surveys and Tutorials. 16, 3 (2014), 1658–1686.
[4] Patel, M. and Wang, J. 2010. APPLICATIONS, CHALLENGES, AND PROSPECTIVE IN EMERGING BODY AREA NETWORKING TECHNOLOGIES. IEEE Wireless Communications. 17, 1 (2010).
[5] Starner, T. 2001. The Challenges of Wearable Computing: Part 2. IEEE Micro. 21, (2001), 54–67.
[6] Ullah, S., Higgins, H., Braem, B., Latre, B., Blondia, C., Moerman, I., Saleem, S., Rahman, Z. and Kwak, K.S. 2012. A comprehensive survey of wireless body area networks on PHY, MAC, and network layers solutions. Journal of Medical Systems. 36, 3 (2012), 1065–1094.
Design Considerations for Garment Manufacturing
- Wires and leads should be incorporated into seams when possible.
- Wires and leads should almost always run vertical (up and down) the body and not horizontal (around) the body
- Look to fabric manipulation, old world textile techniques, and couture sewing techniques as inspiration for designing electronic textile fabric interfaces and sensors.
Accessibility Considerations for Garment Manufacturing
- Some garment are specifically designed to be donned and doffed by people with mobility issues [4, 5]. Designing wearable systems for incorporation with these garments should follow the same strategies as any other garment. However if redesigning seams and closures to afford the wearable technology incorporation it is important not to impede the donning and doffing functionality of the accessible garment.
[1] Jones, S.J. 2005. Fashion Design. Watson-Guptill Publications.
[2] Joseph-Armstrong, H. 2000. Pattern Making for Fashion Design. Prentice Hall.
[3] Shaeffer, C.B. 2001. Couture Sewing Techniques. The Taunton Press.
[4] Watkins, S.M. 1995. Clothing: The Portable Environment. Iowa State Press.
[5] Watkins, S.M. and Dunne, L.E. 2015. Functional Clothing Design: From Sportswear to Spacesuits. Bloomsbury Publishing.
[6] Wolff, C. 1996. The Art of Manipulating Fabric. Krause Publications.
Design Considerations for Social Acceptability
- Body placement of wearable technology can drastically affect the social acceptability of the wearable device. In general avoid touch-based interactions and displays within regions of the body associated with sex or elimination of body waste. An exception would be if the wearable device is specifically designed to aid in sexual stimulation.
- In general it is also advisable to avoid the breast and an interaction location for wearable technology (except for wearable devices specific to cis-gender males, but there are still more socially acceptable places on the body which could work better). An exception would be products designed to work with the breast (e.g. a breast milk pump).
Accessibility Considerations for Social Acceptability
- Sometimes users want assistive technology to be conspicuous so that others know about their needs. Other times users want assistive technology to be inconspicuous so they can go about their daily life without a disability being the focus. Designers should work with users to allow for wearing technology in ways that can throttle the visibility of wearable assistive technology.
- Wearable assistive technology should conform to the same social acceptability standards as other wearable technology. Assistive devices do not have to look like medical devices.
[1] Dunne, L., Profita, H. and Zeagler, C. 2014. Social Aspects of Wearability and Interaction. Wearable Sensors: Fundamentals, Implementation and Applications. E. Sazonov and M. Neuman, eds. Elsevier Inc. 25–43.
[2] Dunne, L.E., Profita, H., Zeagler, C., Clawson, J., Gilliland, S., Do, E.Y. i L. and Budd, J. 2014. The social comfort of wearable technology and gestural interaction. Annual International Conference of the IEEE Engineering in Medicine and Biology Society. I (2014), 4159–4162.
[3] Goffman, E. 1959. The presentation of self in everyday life.
[4] Profita, H., Clawson, J., Gilliland, S., Zeagler, C., Starner, T., Budd, J. and Do, E.Y.-L. 2013. Don’t mind me touching my wrist: a case study of interacting with on-body technology in public. Proceedings of the 17th annual International symposium on wearable computers – ISWC ’13. (2013), 89–96.
[5] Starner, T., Rhodes, B. and Weaver, J. 1999. Everyday-use Wearable Computers. Georgia Tech Technical Report.
[6] Zeagler, C., Starner, T., Hall, T. and Wong Sala, M. 2015. Meeting the Challenge: The Path Towards a Consumer Wearable Computer. Georgia Institute of Technology.
By combining the most frequent reasons for wearable technology, and functional, technical, and social considerations in on-body location with the affordances offered to technology by different locations on the body, a map of current on-body locations for wearable technology can be developed. The darker the green the more likely a device should be placed on the body in the location.